The cosmos is a vast and mysterious realm, filled with celestial wonders that stretch the imagination and challenge our understanding of the universe.
Among these captivating phenomena are quasars—luminous beacons of energy that outshine entire galaxies and provide a glimpse into the distant past of our universe. As some of the brightest objects in the cosmos, quasars are fueled by supermassive black holes at the centers of galaxies, emitting tremendous amounts of light and radiation as they consume surrounding matter. In this blog post, we will embark on an exhilarating journey to explore the different types of quasars, delving into their unique characteristics, behaviors, and the role they play in the grand tapestry of cosmic evolution. Whether you are an aspiring astronomer or simply a curious soul captivated by the mysteries of space, join us as we unveil the secrets of these extraordinary cosmic entities and their significance in our quest to understand the universe.
1. Introduction to Quasars: What Are They?
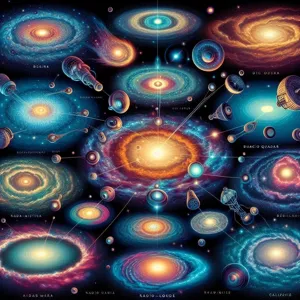
Quasars, short for “quasi-stellar objects,” are among the most fascinating and enigmatic entities in the universe. They are powered by supermassive black holes at the centers of distant galaxies, where immense gravitational forces create a cauldron of energy and light. As matter spirals into these black holes, it forms an accretion disk—a swirling mass of hot gas and dust that emits powerful radiation across the electromagnetic spectrum. This process can unleash energy equivalent to that of hundreds of galaxies combined, making quasars some of the brightest objects in the cosmos.
Discovered in the 1960s, quasars were initially perplexing to astronomers who observed their extraordinary luminosity and peculiar spectral lines. What set them apart was their staggering distances from Earth; many are located billions of light-years away, meaning we are observing them as they were in the early universe. This characteristic provides invaluable insights into the formation and evolution of galaxies over cosmic time.
The sheer brightness of quasars allows them to outshine their host galaxies, making them visible even at incredible distances. They serve as beacons, illuminating the vast expanse of space and offering clues about the conditions of the early universe. As we delve deeper into the world of quasars, we uncover not only the mechanics behind their brilliance but also their significance in understanding the cosmos and our place within it. In the following sections, we will explore the different types of quasars and the roles they play in the grand tapestry of the universe.
2. The History of Quasar Discovery
The journey of discovering quasars is a fascinating tale of astronomical exploration that has revolutionized our understanding of the universe. It all began in the 1960s when astronomers were puzzled by a series of faint radio sources detected by radio telescopes. These objects emitted vast amounts of energy yet were located at great distances, leading to questions about their true nature.
The term “quasar,” short for “quasi-stellar radio source,” was first coined in 1964 by a team of astronomers, including Maarten Schmidt, who played a pivotal role in unraveling the mystery. Schmidt’s groundbreaking work involved identifying the first quasar, 3C 273, which was later found to be located over 2 billion light-years away in the constellation Virgo. This discovery was monumental; it not only confirmed that quasars were indeed distant galaxies but also provided evidence for the existence of massive black holes at their centers.
As research progressed, it became clear that quasars were not isolated phenomena. By the late 1970s, hundreds of quasars had been cataloged, all exhibiting similar characteristics: extraordinary luminosity, rapid variability, and significant redshifts in their spectral lines. These features indicated that quasars were not just distant stars but powerful, energetic entities resulting from the accretion of material into supermassive black holes.
Over the decades, advancements in technology, such as the development of more sensitive telescopes and observational techniques, have enabled astronomers to study quasars in greater detail. Today, quasars are recognized as some of the brightest and most energetic objects in the universe, serving as crucial beacons for understanding cosmic evolution, the formation of galaxies, and the behavior of dark matter. Their discovery not only expanded the boundaries of our universe but also opened new avenues of inquiry into the fundamental nature of space and time. As we continue to explore the cosmos, the legacy of quasar research remains a testament to the power of curiosity and the unrelenting quest for knowledge.
3. Understanding the Structure of Quasars
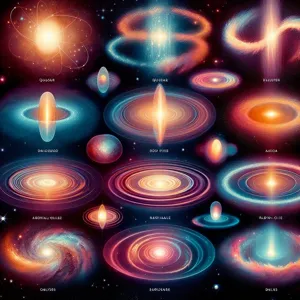
Quasars, or quasi-stellar objects, are among the most fascinating and enigmatic entities in the universe, showcasing a complex structure that contributes to their extraordinary luminosity and behavior. At their core, quasars are powered by supermassive black holes, often millions to billions of times the mass of our Sun. These black holes, lurking at the centers of distant galaxies, create an intense gravitational pull that attracts surrounding gas and dust. As this material spirals inward, it forms an accretion disk—an intricate structure of swirling matter that generates immense heat and light as friction and gravitational forces work their magic.
The energy produced in this accretion process is what makes quasars shine so brightly, often outshining entire galaxies. The temperature of the accretion disk can reach millions of degrees, emitting radiation across the electromagnetic spectrum, from radio waves to X-rays. This emission can be so powerful that it allows quasars to be observed from billions of light-years away, giving astronomers a glimpse into the early universe.
Surrounding this central region is a structure known as the broad-line region, where fast-moving clouds of gas emit broad spectral lines due to their high velocities. These clouds are responsible for the characteristic spectral features that allow scientists to classify quasars and determine their distances and properties. Beyond this lies the narrow-line region, composed of slower-moving gas that produces narrower spectral lines. Together, these components create a dynamic and highly energetic environment that characterizes quasars.
Understanding the structure of quasars not only reveals the mechanics of their immense energy production but also provides insights into the evolution of galaxies and the universe itself. As researchers continue to study these cosmic beacons, they unlock the mysteries of black holes, galaxy formation, and the very fabric of space-time. Each quasar serves as a cosmic lighthouse, illuminating our understanding of the cosmos and inviting us to explore the depths of the universe with renewed curiosity.
4. Types of Quasars: An Overview
When delving into the enigmatic realm of quasars, it’s essential to understand that these cosmic entities aren’t a monolithic group; rather, they exhibit a fascinating diversity that can be categorized into several types based on their properties and behaviors.
**1. Radio-Loud Quasars:** These quasars are characterized by their strong emission of radio waves, making them some of the brightest objects in the universe. They often possess powerful jets that can stretch across vast distances, ejecting particles at nearly the speed of light. The radiation from these jets can outshine the light from the quasar itself, providing valuable insights into the physics of black holes and the environments surrounding them.
**2. Radio-Quiet Quasars:** In contrast to their radio-loud counterparts, radio-quiet quasars emit very little radio energy. Instead, their luminosity is primarily in the optical and ultraviolet wavelengths. These quasars are more numerous and may represent a later evolutionary stage of quasar activity, where the intense outflows have settled down, leading to a more subdued energy profile.
**3. Broad-Line Quasars:** These quasars display broad emission lines in their spectra, indicating the presence of gas moving at high speeds near the black hole. The broad lines are a result of the Doppler effect, as gas clouds orbit the supermassive black hole at incredible velocities. This category allows astronomers to infer the mass of the black hole and the dynamics of the surrounding accretion disk.
**4. Narrow-Line Quasars:** In contrast, narrow-line quasars exhibit narrower emission lines, suggesting a more orderly motion of gas. These quasars are often associated with less turbulent environments and can provide a different perspective on the processes occurring near supermassive black holes.
**5. Distant Quasars:** These quasars are located at vast distances from Earth, allowing astronomers to study the early universe. Their light has traveled billions of years to reach us, offering a glimpse into the conditions of the cosmos when galaxies were still forming. Distant quasars are critical for understanding the evolution of galaxies and the growth of black holes over cosmic time.
Exploring these different types of quasars not only enriches our understanding of the universe but also highlights the complex interactions between black holes and their surroundings. Each type offers a unique window into the mechanisms driving the most energetic phenomena in the cosmos, making quasars a captivating subject of study for astronomers and cosmic enthusiasts alike.
5. Radio-loud vs. Radio-quiet Quasars
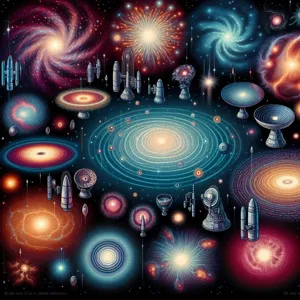
When delving into the enigmatic realm of quasars, one of the most fascinating distinctions we encounter is the division between radio-loud and radio-quiet quasars. These two categories not only highlight the diversity of these celestial phenomena but also offer insights into the underlying mechanisms that drive their incredible luminosity and behavior.
**Radio-loud quasars** are the more boisterous of the two, emitting powerful radio waves that can be detected across vast distances. Their energetic jets, which can extend thousands of light-years into space, are a byproduct of the intense gravitational forces at play around supermassive black holes at their centers. These quasars are characterized by their broad emission lines and are often surrounded by extensive structures known as radio lobes. These lobes can be seen as beacons of energy, illuminating the intergalactic medium and providing crucial clues about the quasar’s environment and the processes occurring within it. The most famous example of a radio-loud quasar is 3C 273, one of the first quasars ever identified, which has long been a subject of study for its impressive radio emissions.
In contrast, **radio-quiet quasars** exhibit a more subdued presence in the radio spectrum. While they still shine brightly in optical and ultraviolet wavelengths, their radio emissions are minimal or nearly absent. This quiet nature does not diminish their importance; in fact, radio-quiet quasars are believed to represent the majority of all quasars, and their study is essential for understanding the broader population of active galactic nuclei. These quasars typically have narrower emission lines, indicating less energetic processes at work compared to their radio-loud counterparts. The differences in their emissions suggest varying accretion rates and orientations of the surrounding material, leading to distinct evolutionary paths for these galaxies.
Understanding the dichotomy between radio-loud and radio-quiet quasars not only enriches our comprehension of these extraordinary objects but also sheds light on the complex interplay between black holes and their host galaxies. As astronomers continue to explore these cosmic phenomena, the duality of quasars serves as a reminder of the vast and intricate tapestry of the universe, inviting us to ponder the mysteries that lie beyond our own galaxy.
6. Broad Absorption Line Quasars (BALs)
Broad Absorption Line Quasars, commonly referred to as BALs, are a fascinating subset of quasars that reveal the dynamic and complex nature of the universe. Characterized by their unique spectral features, BALs exhibit broad absorption lines in their optical and ultraviolet spectra, which signify the presence of fast-moving gas clouds surrounding the quasar. These gas clouds, composed primarily of ionized metals, are ejected at velocities that can reach up to 20% of the speed of light, creating a mesmerizing cosmic dance that intrigues astronomers.
The distinctive absorption lines found in BALs are indicative of the material being accelerated away from the quasar itself. This phenomenon occurs when the intense radiation emitted by the quasar interacts with the surrounding gas, leading to ionization and the subsequent formation of these broad lines. The presence of such outflows not only provides insight into the quasar’s feeding habits and the growth of supermassive black holes but also offers clues about the impact of quasars on their host galaxies and the intergalactic medium.
Interestingly, BALs are relatively rare compared to their non-BAL counterparts, comprising roughly 10-20% of all known quasars. Their rarity, combined with their unique characteristics, makes them a subject of great interest in the field of astrophysics. Researchers continue to study these cosmic beacons to better understand the physical processes at play, including the mechanisms behind the outflows and how they influence star formation in the surrounding environment.
In summary, Broad Absorption Line Quasars stand as a testament to the intricate tapestry of the cosmos, revealing the interplay between light, gas, and gravity. As we delve deeper into their mysteries, we gain not only a better understanding of quasars themselves but also of the broader processes that govern the evolution of the universe.
7. Narrow-line Seyfert 1 Galaxies (NLS1s)
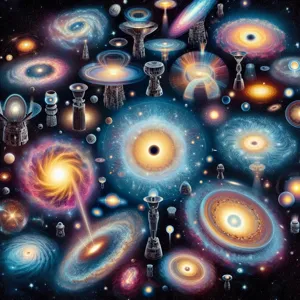
Narrow-line Seyfert 1 Galaxies (NLS1s) are a fascinating subset of quasars that offer unique insights into the complexities of active galactic nuclei. These celestial wonders are characterized by their relatively narrow emission lines in their spectra, which sets them apart from their broader-lined counterparts. This distinct feature is a result of their relatively low black hole masses and high accretion rates, providing a glimpse into the dynamic processes occurring in their cores.
Found predominantly at the lower end of the luminosity scale, NLS1s are often distinguished by their rapid variability and strong ultraviolet and X-ray emissions. These galaxies typically host supermassive black holes that range from hundreds of thousands to a few million solar masses, but despite their lesser mass compared to other Seyfert galaxies, they exhibit extreme energetic phenomena. Their luminosity can fluctuate significantly over short timescales, sometimes within just a few days, offering astronomers a perfect laboratory for studying the physics of accretion and relativistic jets.
One of the most intriguing aspects of NLS1s is their propensity to emit powerful outflows and jets, which can rival those of more massive quasars. These outflows can impact their host galaxies, influencing star formation and the overall evolution of the galactic environment. Additionally, some NLS1s have been associated with various forms of activity, including gamma-ray emissions, further blurring the lines of classification within active galaxies.
The study of NLS1s not only enriches our understanding of quasars but also sheds light on the evolutionary pathways of galaxies. Their presence in the local universe indicates that supermassive black holes can grow rapidly and become highly active even in less massive systems, challenging previous notions about the relationship between black hole mass and quasar activity. As research continues to evolve, NLS1s remain a captivating subject for astronomers striving to unravel the mysteries of the cosmos.
8. The Role of Supermassive Black Holes in Quasars
Supermassive black holes are the enigmatic powerhouses at the heart of quasars, playing a crucial role in their formation and behavior. These colossal entities, with masses millions or even billions of times greater than that of our Sun, possess a gravitational pull so strong that not even light can escape once it crosses the event horizon. At the core of a quasar, the supermassive black hole acts as a cosmic vacuum cleaner, drawing in gas, dust, and other stellar materials from its surroundings.
As matter spirals into the black hole, it forms an accretion disk—an intense whirlpool of hot, luminous gas that radiates energy across the electromagnetic spectrum. This process generates staggering amounts of light, making quasars some of the brightest objects in the universe. In fact, a single quasar can emit more light than an entire galaxy, illuminating the vast cosmic void around it.
The immense energy output from these accretion disks is the result of gravitational forces converting potential energy into kinetic energy, heating the infalling material to millions of degrees. This extreme heating causes the gas to emit radiation, producing not just visible light but also ultraviolet, X-ray, and radio waves. As a result, quasars serve as beacons, allowing astronomers to probe the distant reaches of the universe and gain insight into the behavior of matter in extreme gravitational fields.
Moreover, the relationship between quasars and their supermassive black holes is complex and dynamic. When a quasar is active, it can influence its host galaxy’s evolution, regulating star formation and altering the distribution of matter within it. This interplay between the supermassive black hole and its environment underscores the vital role quasars play in our understanding of cosmic evolution.
In summary, supermassive black holes are not just the engines driving the brilliance of quasars; they are fundamental to our grasp of the universe’s history and structure. By studying these fascinating phenomena, we unlock the secrets of the cosmos, illuminating the pathways of galactic formation and the intricate dance of gravity and light that shapes our vast universe.
9. Quasars and Their Host Galaxies
Quasars, or quasi-stellar objects, are not only fascinating in their own right but also provide a compelling glimpse into the dynamic relationship they share with their host galaxies. These extraordinarily luminous entities, powered by supermassive black holes at their centers, are typically located at the heart of galaxies that are undergoing intense periods of star formation and evolution.
When we observe quasars, we are witnessing the radiant light emitted from the accretion disk surrounding these black holes. This light can outshine entire galaxies, allowing astronomers to detect quasars at vast distances—often billions of light-years away—making them crucial tools for studying the early universe. Each quasar is a beacon, illuminating the characteristics of its host galaxy, which may otherwise remain hidden in the cosmic depths.
The host galaxies of quasars often exhibit unique features that reflect their tumultuous environments. Many are massive elliptical galaxies, characterized by their older stellar populations and a rich history of galactic interactions and mergers. These interactions can funnel gas and dust into the center, feeding the supermassive black hole and igniting the quasar’s brilliance. In contrast, some quasars reside in spiral galaxies, where ongoing star formation contributes to vibrant stellar activity.
The relationship between quasars and their host galaxies is a two-way street. As quasars emit tremendous amounts of energy, they can influence their surroundings, driving outflows of gas and regulating star formation rates within their galaxies. This feedback mechanism plays a pivotal role in galaxy evolution, affecting everything from the growth of the galaxy itself to the formation of new stars.
Studying quasars and their host galaxies not only helps us understand these enigmatic objects but also offers insights into the larger processes governing the universe. As we delve deeper into the nature of quasars, we begin to unravel the intricate tapestry of cosmic evolution, revealing how these luminous beacons are intertwined with the galaxies that house them.
10. The Importance of Quasars in Cosmology
Quasars are not just cosmic curiosities; they hold a pivotal role in the field of cosmology, offering invaluable insights into the universe’s early stages and the formation of galaxies. As some of the most luminous and distant objects in the universe, quasars serve as beacons that illuminate our understanding of cosmic evolution.
One of the key reasons quasars are so important is their ability to act as distant lighthouses, helping astronomers probe the vastness of space. Because quasars are powered by supermassive black holes at the centers of galaxies, their immense brightness allows scientists to observe phenomena billions of light-years away. This means that when we study quasars, we are effectively looking back in time, gaining a glimpse into the universe as it was when these objects first emitted their light. This capability enables researchers to investigate the conditions of the early universe, including the formation and growth of galaxies.
Additionally, quasars contribute to our understanding of dark matter and dark energy, the mysterious forces that make up a significant portion of the universe. By studying the gravitational effects of quasars on their surroundings, scientists can gather clues about the distribution of dark matter in the cosmos. This research can potentially lead to groundbreaking discoveries about the fundamental components of the universe and how they interact.
Moreover, quasars are instrumental in refining our models of cosmic structure formation. They help astronomers understand how galaxies evolve over time and how supermassive black holes influence their host galaxies. The relationship between quasars and their surrounding environments provides crucial data on the processes that govern galaxy formation and development.
In summary, quasars are more than just fascinating astronomical objects; they are keys to unlocking the mysteries of the universe. Their significance in cosmology cannot be overstated, as they offer a unique window into the past, contribute to our understanding of dark matter and energy, and enhance our knowledge of galaxy formation. As we continue to explore these enigmatic entities, we take one step closer to unraveling the complexities of the cosmos.
11. Challenges in Studying Quasars
Studying quasars, those enigmatic beacons of light from the distant universe, presents a myriad of challenges that researchers must navigate. First and foremost is their incredible distance from Earth. Quasars are among the most luminous objects in the cosmos, often found billions of light-years away, which means that the light we see today has taken an immense amount of time to reach us. This vast expanse of space and time complicates our understanding of their properties and behaviors, as the further away we look, the earlier in the universe’s history we are observing.
Moreover, quasars are often shrouded by clouds of gas and dust, which can obscure our view and affect the data we collect. This interstellar material can absorb and scatter light, making it difficult to accurately measure the quasar’s brightness and spectrum. Astronomers rely on advanced imaging techniques and powerful telescopes, such as the Hubble Space Telescope and the upcoming James Webb Space Telescope, to penetrate these cosmic veils and gather clearer data.
Another significant challenge lies in the variability of quasars themselves. These objects are not static; they change in brightness over time scales that can range from days to years. This variability complicates the analysis of their physical characteristics and the mechanisms driving their emissions. Temporal observations must be meticulously planned and executed to capture these changes, requiring a coordinated effort across different observatories worldwide.
Finally, the sheer variety of quasars adds another layer of complexity to their study. With different types categorized by their emission features, such as radio-loud and radio-quiet quasars, astrophysicists must consider a wide range of theoretical models to explain their formation and evolution. The interplay between supermassive black holes at their centers, the accretion of surrounding material, and the effects of their environments create a puzzle that continues to captivate scientists.
In summary, while the study of quasars offers remarkable insights into the early universe and the fundamental workings of black holes, it is fraught with challenges that require innovative approaches, cutting-edge technology, and a collaborative spirit among astronomers across the globe. Each new discovery not only enhances our understanding of these cosmic giants but also propels us further into the mysteries of the universe itself.
12. Recent Discoveries and Advances in Quasar Research
The realm of quasar research is an ever-evolving frontier, marked by groundbreaking discoveries and technological advancements that continuously reshape our understanding of these cosmic phenomena. Recent years have witnessed a surge in interest and exploration, driven by sophisticated telescopes and innovative observational techniques. For instance, the advent of the James Webb Space Telescope (JWST) has opened new avenues for studying quasars, allowing astronomers to peer deeper into the universe than ever before.
One of the most exciting recent discoveries involves the identification of distant quasars that illuminate the early universe, providing invaluable insights into the conditions that prevailed shortly after the Big Bang. These ancient quasars, some dating back over 13 billion years, serve as time capsules, enabling scientists to investigate the formation of galaxies and the evolution of supermassive black holes during a pivotal era in cosmic history.
Moreover, advances in spectroscopy have allowed researchers to analyze the light emitted by quasars with unprecedented precision. This has led to revelations about their chemical composition, including the presence of heavy elements such as iron and carbon. Understanding the abundance of these elements helps astronomers unravel the processes of star formation and the dynamics within their host galaxies.
Additionally, recent studies have challenged conventional theories regarding the relationship between quasars and their host galaxies. Researchers are exploring how feedback from quasar activity influences star formation rates, challenging the long-held notion that these luminous entities solely grow through accretion of surrounding gas. Instead, findings suggest a more complex interplay, where quasars can regulate their environments, shaping the evolution of their galaxies in profound ways.
As we stand on the cusp of new discoveries, the field of quasar research continues to flourish, fueled by a blend of theoretical advancements and technological progress. Each new finding not only deepens our comprehension of these enigmatic objects but also ignites curiosity about the broader universe, reminding us of the intricate and dynamic tapestry of cosmic evolution that surrounds us.
13. Quasars and the Evolution of the Universe
Quasars, or quasi-stellar objects, are not just celestial phenomena; they are beacons of the universe’s evolution, illuminating our understanding of cosmic history. Formed in the early universe, these incredibly luminous objects are powered by supermassive black holes at the centers of distant galaxies. As matter spirals into these black holes, it emits vast amounts of energy, resulting in the intense brightness that can outshine entire galaxies. This luminosity allows astronomers to observe quasars from billions of light-years away, effectively serving as time machines that transport us back to the universe’s formative years.
The study of quasars has provided crucial insights into the structure and development of the cosmos. Their incredible distances and ages help us piece together the timeline of the universe, revealing how galaxies evolved and interacted over billions of years. By analyzing the light emitted from these objects, scientists can infer the conditions of the early universe, including the rate of star formation and the distribution of dark matter.
Moreover, quasars play a pivotal role in understanding the expansion of the universe. Their brightness and specific light signatures allow researchers to measure cosmic distances with great precision, contributing to our knowledge of how the universe has expanded since the Big Bang. The existence of quasars also raises intriguing questions about galaxy formation; they suggest that supermassive black holes may be a common feature of galaxies and that their growth is intricately linked to the evolution of their host galaxies.
In essence, quasars are more than just distant points of light; they are vital clues that help us unravel the complex narrative of the universe’s evolution, offering glimpses into a time when the cosmos was still young and dynamic. As we continue to explore these extraordinary objects, we edge closer to understanding the fundamental processes that shaped the universe we inhabit today.
14. Future Directions in Quasar Observation
As we stand on the brink of a new era in astrophysics, the future of quasar observation holds immense promise, driven by cutting-edge technology and innovative methodologies. With the advent of next-generation telescopes and observational techniques, scientists are poised to deepen their understanding of these enigmatic celestial objects. One of the most exciting developments is the James Webb Space Telescope (JWST), which promises to provide unprecedented views of the early universe, allowing researchers to study quasars in their infancy, potentially unraveling the mysteries of black hole formation and galaxy evolution.
Moreover, advancements in radio astronomy, particularly through facilities like the Square Kilometre Array (SKA), will enable astronomers to detect quasars that are currently too faint to observe with existing technology. By capturing the radio emissions from these distant objects, the SKA will help map the distribution of quasars across different cosmic epochs, offering insights into the interplay between dark matter, dark energy, and the formation of large-scale structures in the universe.
In addition to these technological breakthroughs, collaborative international efforts, such as the Vera C. Rubin Observatory’s Legacy Survey of Space and Time (LSST), will revolutionize our approach to quasar observation. By conducting wide-field surveys and monitoring the sky continuously, LSST will uncover transient phenomena associated with quasars, shedding light on their variability and providing a richer context for understanding their behavior.
As we explore these future directions, the integration of machine learning and artificial intelligence into astronomical research holds the potential to expedite the analysis of vast datasets generated by these new observatories. By automating the identification of quasar candidates and predicting their properties, researchers can focus their efforts on the most promising targets, accelerating our quest to decipher the complex narratives woven into the fabric of the cosmos.
The horizon of quasar research is bright, and with each new observational advancement, we inch closer to unraveling the intricate tapestry of our universe’s history—one quasar at a time. The future is not merely about observing these distant beacons of light; it’s about understanding their role in the grand cosmic ballet and the profound implications they hold for the nature of matter, energy, and the very fabric of spacetime itself.
15. Conclusion: The Significance of Quasars in Understanding the Cosmos
In conclusion, quasars represent one of the most fascinating and enigmatic phenomena in the universe, serving as beacons of light that illuminate our understanding of cosmic evolution. These incredibly luminous objects, powered by supermassive black holes at the centers of distant galaxies, offer insights into the early universe and the formation of large-scale structures. By studying quasars, astronomers can glean crucial information about the distribution of matter, the nature of dark energy, and the complex interplay of forces that govern the cosmos.
The significance of quasars extends beyond mere astronomical curiosities; they are essential to our quest for knowledge about the universe’s history, structure, and the fundamental laws that govern it. Their extreme brightness allows us to observe galaxies that existed billions of years ago, providing a glimpse into a time when the universe was still in its infancy. This ability to peer back in time enables scientists to formulate and test theories about the evolution of galaxies, the formation of stars, and the behavior of black holes.
Moreover, quasars challenge our understanding of physics, particularly in the realms of relativity and quantum mechanics. The intense gravitational forces and rapid rotational speeds associated with these phenomena push the limits of our current theories, inspiring new research and innovative ideas that can lead to groundbreaking discoveries.
As we continue to explore the cosmos, quasars will undoubtedly remain a focal point of astronomical research. Their study not only enriches our comprehension of the universe but also ignites a sense of wonder about our place within it. Each quasar is a cosmic lighthouse, guiding us through the vast and mysterious expanse of space, reminding us that there is still so much more to learn and discover.
As we conclude our journey through the fascinating world of quasars, it’s clear that these celestial powerhouses hold the key to understanding the universe’s evolution and the complex processes at play in distant galaxies. From the brilliant luminosity of radio-loud quasars to the enigmatic nature of their radio-quiet counterparts, each type offers a unique glimpse into the cosmos and invites us to ponder the mysteries beyond our reach. Armed with this newfound knowledge, we encourage you to continue exploring the wonders of the universe, whether through books, documentaries, or even stargazing nights. The cosmos is vast and full of surprises, and with each discovery, we inch closer to unraveling the grand tapestry of existence. Thank you for joining us on this cosmic adventure—may your curiosity always lead you to the stars!